In the realm of modern technology, pulsed lasers are revolutionizing industries by enabling precise and efficient material processing. You might be wondering how to choose the right materials for various laser wavelengths to maximize their potential. With over 75% of manufacturers relying on laser technology for cutting and engraving, understanding the unique interactions between different wavelengths and materials is crucial for achieving optimal results.
This article promises to guide you through the suitable materials for each wavelength of pulsed lasers, highlighting their specific advantages and applications. From ultraviolet lasers used in microelectronics to femtosecond lasers in medical procedures, we’ll explore how these technologies can enhance your projects. Get ready to unlock the secrets of laser-material interactions and discover how you can leverage this knowledge for your own innovative applications!
What’s Wavelengths of Pulsed Lasers
Wavelengths of pulsed lasers refer to the specific lengths of light waves emitted during the laser’s operation. The wavelength is a critical parameter that influences how the laser interacts with different materials. It is measured in nanometers (nm), and different wavelengths correspond to different colors and types of light, from ultraviolet through visible to infrared.For instance, common pulsed laser wavelengths include:
- 355 nm: Ultraviolet light, often used in medical and cosmetic procedures.
- 532 nm: Green light, widely used in laser pointers and some medical applications.
- 1064 nm: Near-infrared light, commonly used in industrial applications and for deeper tissue penetration in medical treatments.
Understanding these wavelengths helps determine the laser’s effectiveness for specific tasks, such as cutting materials or performing delicate surgical procedures.
Ultraviolet (UV) Lasers (Below 400 nm)
Ultraviolet (UV) lasers, operating in the wavelength range below 400 nm, are crucial in various applications due to their unique properties. These lasers can be categorized into three segments: Near Ultraviolet (NUV, 315-400 nm), Middle Ultraviolet (MUV, 200-315 nm), and Far Ultraviolet (FUV, 100-200 nm). Each segment serves specific purposes across industries such as microelectronics, medical diagnostics, and material processing. For instance, UV lasers are vital for microfabrication, enabling the production of intricate components in integrated circuits and microelectromechanical systems (MEMS).
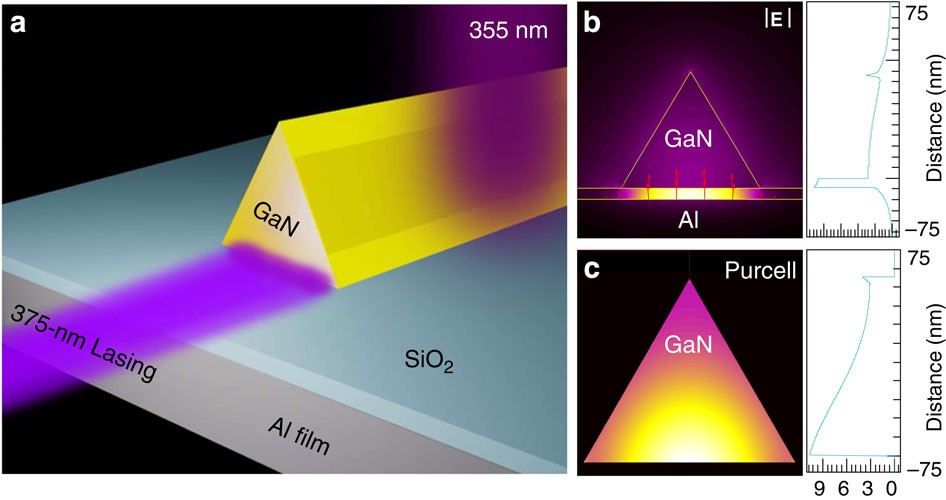
Applications of UV Lasers
UV lasers are extensively utilized in microfabrication, where their ability to create fine features is essential for manufacturing advanced electronic devices. In the medical field, these lasers are employed for phototherapy and surgical procedures, particularly in dermatology for conditions such as psoriasis. Additionally, they play a crucial role in disinfection technologies, leveraging their germicidal properties to eliminate pathogens effectively.
Advantages of UV Lasers
The advantages of UV lasers stem from their high absorption rates across various materials, facilitating efficient material removal through photochemical processes. Their precision processing capabilities make them ideal for applications requiring high-resolution features. Furthermore, the short pulse duration minimizes thermal damage to sensitive materials, preserving their integrity during processing. This combination of precision and minimal thermal impact positions UV lasers as a preferred choice in many industrial applications.
Materials Processed by UV Lasers
Different materials respond uniquely to UV laser processing:
- Polymers: Commonly used polymers such as PMMA (Polymethyl methacrylate), polycarbonate, and epoxy resins can be effectively ablated or modified.
- Biomaterials: Hydrogels and biological tissues are processed with precision using UV lasers, enabling advancements in bioprinting and tissue engineering.
- Metals: Thin layers of metals like gold or silver can be precisely ablated without affecting underlying structures.
Challenges and Innovations
Despite their advantages, developing efficient UV laser systems presents challenges. Achieving high output power while maintaining low threshold currents is crucial for advancing UV laser diodes. Recent innovations include GaN-based semiconductor lasers that promise lower thresholds and improved efficiency in the UV range. These developments pave the way for more compact and cost-effective UV laser solutions suitable for a broader range of applications.
UV lasers below 400 nm represent a critical technology with diverse applications across multiple sectors. Their unique properties enable precise material processing while minimizing thermal damage, making them indispensable tools in modern manufacturing and medical practices. As technology advances through 2025 and beyond, the efficiency and accessibility of these lasers are expected to improve further, expanding their potential uses.
Visible Lasers (400 nm – 700 nm)
Visible lasers operate within the 400 nm to 700 nm wavelength range, which is critical for various applications in medicine, manufacturing, and consumer electronics. This spectrum corresponds to the visible light that humans can perceive, with colors transitioning from violet at 400 nm to red at 700 nm. Understanding the properties and applications of these lasers is essential for effective use and safety.
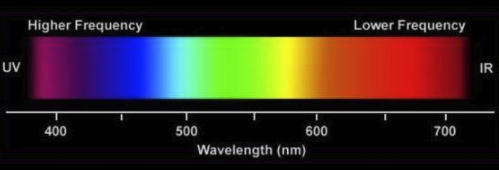
Applications of Visible Lasers
Visible lasers are utilized across diverse fields due to their versatility:
- Medical Treatments: These lasers are integral in procedures like laser hair removal and skin rejuvenation, allowing for targeted treatments with minimal damage to surrounding tissues.
- Industrial Manufacturing: In manufacturing, they excel in cutting and engraving materials, providing high visual contrast for detailed applications.
- Consumer Electronics: Commonly found in devices like laser pointers and optical disc drives, these lasers enhance functionality through clarity and precision.
Safety Considerations
While visible lasers offer significant benefits, they also pose potential hazards. The risk of eye injury is particularly notable since the human eye focuses light directly onto the retina. Therefore, adhering to safety protocols is crucial:
- Laser Classifications: Lasers are categorized based on their potential hazards. For example:
- Class 1: Safe under all conditions.
- Class 2: Safe for brief exposure (up to 0.25 seconds).
- Class 3B and Class 4: Potentially hazardous and require protective eyewear.
Types of Visible Lasers
Several types of visible lasers exist based on their construction and operational principles:
- Laser Diodes: These compact devices emit light through semiconductor materials and are commonly used in consumer electronics.
- Solid-State Lasers: Utilizing solid gain media like ruby or Nd:YAG, these lasers are known for their high power and efficiency.
- Gas Lasers: Helium-neon and argon-ion lasers provide stable output at specific wavelengths.
By grasping the applications, safety considerations, and types of visible lasers within the 400 nm to 700 nm range, users can effectively harness their capabilities while ensuring safe practices. Always prioritize safety by using appropriate protective equipment when working with higher-class lasers.
Near-Infrared (NIR) Lasers (700 nm – 1400 nm)
Near-infrared (NIR) lasers, which operate within the 700 nm to 1400 nm wavelength range, are integral to a variety of applications including medical imaging, industrial processing, and scientific research. These lasers excel in penetrating materials while minimizing reflection issues, making them particularly effective for tasks such as welding and cutting. The unique properties of NIR light allow it to interact with materials in ways that visible light cannot, enhancing processing efficiency and effectiveness.
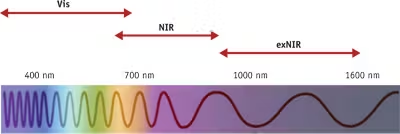
Applications of NIR Lasers
NIR lasers are extensively utilized in medical diagnostics and imaging techniques due to their superior penetration capabilities. For instance, optical-resolution photoacoustic microscopy (OR-PAM) employs NIR light to achieve impressive imaging depths of up to 3.2 mm in biological tissues, such as chicken breast. This non-invasive technique is invaluable for early disease detection and monitoring. Additionally, NIR lasers are used in photodynamic therapy (PDT), where their ability to target specific tissue components with minimal damage enhances treatment outcomes. As technology advances, the range of applications for NIR lasers continues to expand.
Materials Processed with NIR Lasers
The versatility of NIR lasers extends to the types of materials they can effectively process:
- Metals: Common metals like titanium and stainless steel benefit from the lower reflectivity of NIR wavelengths, allowing for efficient cutting and welding.
- Plastics: Various polymers can be processed effectively with NIR lasers, which penetrate deeper into thicker sections compared to other laser types.
This capability not only improves processing efficiency but also reduces the risk of energy loss during operations, making NIR lasers a preferred choice in numerous industrial applications.
Advantages of NIR Lasers
Utilizing NIR lasers offers several significant advantages:
- Good Penetration Depth: The ability of these lasers to penetrate deeper into materials makes them ideal for precise welding or cutting tasks involving thicker sections.
- Reduced Reflection Issues: Compared to longer wavelengths, NIR light exhibits lower reflectivity in metals, enhancing overall processing efficiency.
These benefits underscore the growing importance of near-infrared lasers across various sectors, from manufacturing to healthcare.
Mid-Infrared Lasers (1400 nm – 3000 nm)
Mid-infrared (MIR) lasers, operating within the 1400 nm to 3000 nm wavelength range, are crucial for a variety of applications due to their unique properties. These lasers excel in processing organic materials, such as wood and textiles, owing to their high absorption characteristics. This allows for precise cutting and engraving while minimizing thermal damage. By effectively managing thermal effects during processing, these lasers ensure that even delicate materials maintain their integrity under high-energy exposure.
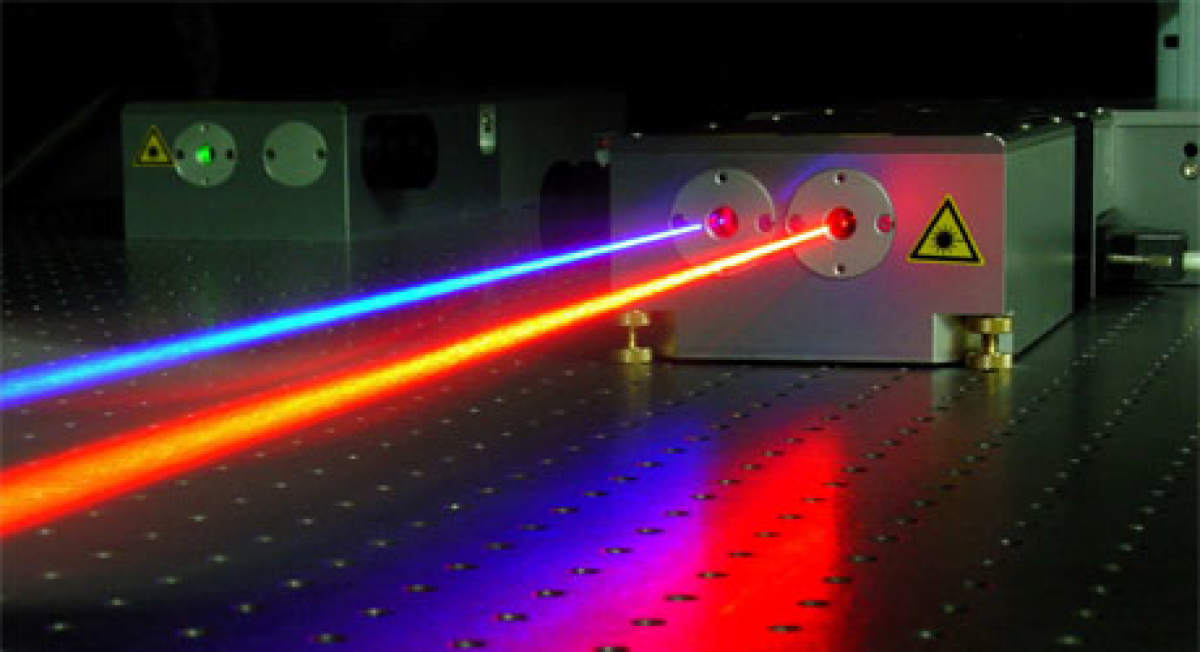
Applications of Mid-Infrared Lasers
- Organic Materials: Mid-infrared lasers are particularly effective for processing organic compounds, enabling efficient cutting and engraving in industries that handle wood and textiles.
- Plastics and Composites: These lasers are also adept at processing plastics and composites, providing high precision in cutting and engraving applications. Their ability to control thermal effects ensures that the structural integrity of materials is preserved throughout the process.
- Medical Applications: In healthcare, mid-infrared lasers facilitate non-invasive diagnostic procedures, such as tissue imaging and laser surgery. Their interaction with biological tissues enhances patient outcomes by allowing for precise treatment without extensive damage.
Advantages of Mid-Infrared Lasers
- High Absorption in Organic Materials: The exceptional absorption characteristics of mid-infrared lasers make them particularly effective for processing organic materials, leading to greater efficiency in cutting and engraving applications.
- Thermal Effects Management: These lasers adeptly manage thermal effects during processing, minimizing the risk of material damage. This feature allows for safe processing of even delicate materials.
The versatility of mid-infrared lasers extends across multiple fields, including environmental sensing, medical diagnostics, and industrial applications. For instance, they are widely used in spectroscopy for detecting greenhouse gases and monitoring soil health. As technology advances, the potential applications of these lasers will likely expand further, enhancing their impact across various industries.
Femtosecond Lasers (Sub-picosecond Pulses)
Femtosecond lasers, which emit light pulses lasting from a few femtoseconds (10⁻¹⁵ seconds) to sub-picoseconds (10⁻¹² seconds), have dramatically advanced various fields by enabling ultra-precise material processing. Their ability to deliver high-energy bursts with minimal thermal damage makes them essential in applications demanding exceptional accuracy. From medical procedures to advanced manufacturing, the impact of these ultrafast lasers is significant and continues to expand through 2025.
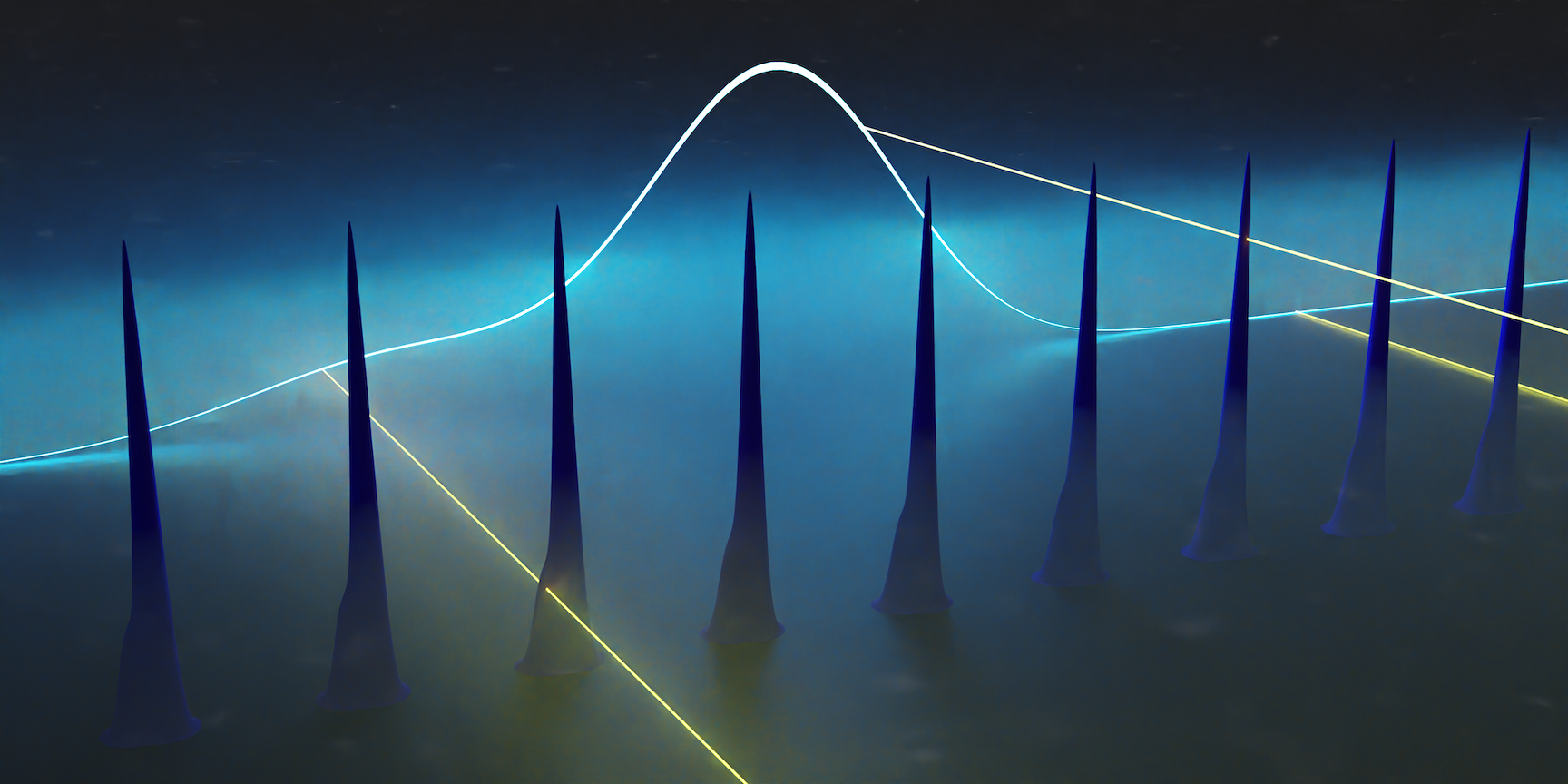
Applications of Femtosecond Lasers
Femtosecond lasers play a crucial role in diverse applications across multiple sectors. In ophthalmology, they are pivotal for LASIK eye surgery, allowing for the creation of precise corneal flaps without the use of blades, which enhances patient safety and reduces recovery times. Additionally, these lasers are utilized in cataract surgery to create accurate incisions in the lens capsule, improving surgical outcomes. Beyond medicine, femtosecond lasers facilitate microfabrication, enabling the construction of intricate structures in materials such as metals and polymers. Their versatility extends to biological applications, where they can manipulate tissues with remarkable precision.
Advantages of Femtosecond Laser Technology
The benefits of femtosecond laser technology are numerous:
- Extreme Precision: The rapid energy delivery minimizes thermal diffusion, allowing for high-precision machining without damaging adjacent materials.
- Material Versatility: These lasers can process a wide array of substances—including metals, ceramics, polymers, and biological tissues—making them suitable for various applications.
- Reduced Heat-Affected Zone: The short pulse duration limits the heat-affected zone during processing, preserving the integrity of the materials involved.
- Enhanced Control: Femtosecond lasers provide precise control over the interaction between the laser and material, allowing for tailored outcomes in micro and nano structuring.
Recent Technological Advancements
Recent innovations in femtosecond laser technology focus on enhancing pulse energy and stability. For instance, advancements such as Active Fiber Loop (AFL) technology allow for versatile GHz burst generation, improving operational flexibility across different regimes. This technology enables tunable pulse durations and has been shown to significantly enhance processing quality. Furthermore, developments in fiber laser systems have resulted in more robust setups that maintain high performance under varying conditions.
Femtosecond lasers are not just limited to industrial applications; their role in medical procedures is expanding. Continuous improvements in femtosecond laser technology drive market growth, particularly in ophthalmic surgeries where precision and safety are paramount. As we move towards 2025, the integration of enhanced imaging systems and software will further solidify their place as essential tools across various fields.
Summary Table of Laser Wavelengths and Suitable Materials
Wavelength Range | Suitable Materials | Key Advantages |
---|---|---|
UV (<400 nm) | Polymers, Biomaterials, Thin Metals | High absorption; precision cutting/engraving |
Visible (400 – 700 nm) | Metals, Glass | Versatile applications in marking and engraving |
NIR (700 – 1400 nm) | Metals, Plastics | Good penetration depth; effective for welding |
Mid-IR (1400 – 3000 nm) | Organic Materials, Plastics | High absorption in organics; reduced thermal damage |
Femtosecond | Almost all materials | Extreme precision; minimal collateral damage |
How Does Wavelengths of Pulsed Lasers Work?
Pulsed lasers are advanced devices that emit light in rapid bursts, enabling precise energy delivery for a variety of applications. The operation begins with energy storage, where the laser medium absorbs energy from an external source, such as a flashlamp or another laser. This stored energy is essential for generating high-intensity light pulses.
Following energy storage, pulse generation occurs through methods like Q-switching and mode-locking. In Q-switching, the quality factor of the laser cavity is temporarily reduced, allowing energy to accumulate before being released in a powerful pulse. Mode-locking synchronizes multiple oscillating modes within the laser cavity, producing extremely short pulses measured in femtoseconds.
The emitted wavelength of the pulse is dictated by the gain medium utilized. For example, a neodymium-doped yttrium aluminum garnet (Nd:YAG) laser typically emits at 1064 nm. This wavelength can be adjusted using techniques such as frequency doubling or tripling, which modify the light’s frequency to suit specific applications.
Pulse duration varies widely, ranging from picoseconds (10^-12 seconds) to milliseconds (10^-3 seconds). This variability influences both peak power and energy delivered per pulse. High peak power is crucial for applications like material ablation, where precise amounts of material can be removed without damaging adjacent areas.
Pulsed lasers function through a series of steps involving energy storage, controlled release via Q-switching or mode-locking, and specific wavelength emission. This operational framework makes them versatile tools across numerous fields, including medical applications and industrial manufacturing.
Key Factors Affecting Laser Wavelengths
The wavelength of a pulsed laser plays a significant role in determining its performance and suitability for various applications. Different wavelengths correspond to varying penetration depths in materials; for instance, a 1064 nm pulsed laser penetrates deeper than a 532 nm laser. This characteristic is vital in fields like dermatology and materials processing, where specific wavelengths are required for optimal results.
Moreover, the relationship between wavelength and pulse duration is critical. Shorter pulse durations generally yield higher peak powers, advantageous for precision tasks such as laser surgery or spectroscopy. By understanding this interplay, researchers and engineers can effectively tailor laser systems to meet specific requirements.
Conclusion
Choosing the right wavelength for pulsed lasers is crucial for optimizing material processing. At OPMT Laser, we recognize the unique challenges you encounter in this field. Each wavelength interacts differently with various materials, enabling you to discover tailored solutions that enhance efficiency across sectors like manufacturing and healthcare. In fact, utilizing the optimal laser wavelength can boost processing speed by up to 30%.
This guide provides essential insights into selecting suitable materials for different wavelengths of pulsed lasers and their advantages. By applying this knowledge, you can elevate your projects with OPMT Laser‘s expertise. Are you prepared to deepen your understanding and explore these advancements further? Let’s embark on this exciting journey together!
Disclaimer
This content is compiled by OPMT Laser based on publicly available information for reference only; mentions of third-party brands and products are for objective comparison and do not imply any commercial association or endorsement.